Integrating the Analysis of Ultrashort-Chain PFAS
Method Development for Simultaneous Analysis of Ultrashort-Chain, Alternative, and Legacy PFAS
Abstract
A simple direct injection LC-MS/MS method was developed and evaluated for the simultaneous analysis of ultrashort-chain, alternative, and legacy per- and polyfluoroalkyl substances (PFAS) in various water samples. This method is recommended for labs wanting to use a single procedure to analyze compounds from all three PFAS categories in potable and non-potable waters.
Introduction
LC-MS/MS methods for the analysis of legacy short-chain (C4, C5) and long-chain (>C5) per- and polyfluoroalkyl substances (PFAS) based on reversed-phase (RP) chromatography are well established. With proper modification, these methods often can also be used for LC-MS/MS analysis of alternative PFAS, such as HFPO-DA (GenX) and ADONA, which are perfluoroalkyl ether carboxylic acids used as PFOA substitutes. Similarly, F-53B is a PFOS alternative produced in China that contains two polyfluoroalkyl ether sulfonate components, 9Cl-PF3ONS and 11Cl-PF3OUdS, which are included as analytes in the updated EPA 537.1 method. However, current LC methods may not be suitable for the analysis of newly trending ultrashort-chain (C2, C3) PFAS, mainly due to their insufficient retention on typical RP columns.
While the use of short-chain PFAS (PFBA and PFBS) is intentional, numerous studies have shown the ubiquitous occurrence of C2 and C3 PFAS in aqueous environmental samples [1,2]. These include trifluoroacetic acid (TFA), perfluoropropanoic acid (PFPrA), perfluoroethane sulfonate (PFEtS), and perfluoropropane sulfonate (PFPrS). It was shown that PFPrA is the predominant PFAS (up to 45% of total detectable PFAS) in rain and snow samples collected from the U.S., France, and Japan [3]. To date, there is no definitive identification of contamination sources and levels for these ultrashort-chain PFAS, but a recent study detected PFEtS and PFPrS in aqueous film-forming foams (AFFFs) and groundwaters from 11 military bases in the U.S. (often used for fire department training exercises) [4], indicating AFFF firefighting foam may be a source of ultrashort-chain PFAS.
Currently, methods that allow the desired combined analysis of ultrashort-chain PFAS with alternative and legacy PFAS are very rare. To fill this gap, we developed a procedure for the simultaneous quantification of a wide range of chain lengths and structures including C3, C4, C8, and alternative PFAS in a variety of water samples.
Experimental
Sample Preparation
In a polypropylene vial, 250 µL water sample aliquots were mixed with 250 µL of 40:60 reagent water:methanol and 5 µL of internal standard solution (5 ng/mL of 13C2-PFHxA, 13C2-PFOA, 13C4-PFOS in methanol). The vial was capped with a polyethylene cap and injected for analysis.
Calibration Standards and Quality Control Samples
Calibration standards were prepared by fortifying reagent water (Optima LC-MS water) with ten PFAS analytes across a range of 5–400 ng/L. The calibration standard solutions were then processed as described in the sample preparation procedure.
Fortified Water Samples
Samples of tap water collected from the Restek facility and three diverse water types (Chicago river water, groundwater, and publicly owned treatment works [POTW] effluent water) supplied by the U.S. EPA were included in this study. Each water type was fortified at 10 (20 ppt for PFPrA) and 80 ppt in duplicate per batch with a total of three batches being prepared and analyzed on different days. Unfortified and fortified water samples were processed through the sample preparation procedure for chromatographic analysis and quantification.
Instrument Conditions
LC-MS/MS analysis of ultrashort-chain PFAS concurrently with alternative and legacy PFAS was performed using a Raptor C18 analytical column and a Shimadzu Nexera X2 HPLC coupled to a SCIEX 4500 MS/MS. A PFAS delay column (cat.# 27854) was installed between the pump mixer and the injector in order to prevent coelution of any instrument-related PFAS with target analytes in the sample. Instrument conditions were as follows and analyte transitions are provided in Table I.
Analytical column: | Raptor C18 2.7 µm, 100 mm x 3.0 mm (cat.# 9304A1E) | |
Delay column: | PFAS delay column (cat.# 27854) | |
Mobile phase A: | 5 mM ammonium acetate in water | |
Mobile phase B: | Methanol | |
Gradient | Time (min) | %B |
0.00 | 20 | |
7.00 | 95 | |
9.00 | 95 | |
9.01 | 20 | |
11.0 | 20 | |
Flow rate: | 0.25 mL/min | |
Run time: | 11 min | |
Injection volume: | 10 µL | |
Column temp.: | 40 °C | |
Ion mode: | Negative ESI | |
Ion spray voltage: | -2000 | |
Source temp.: | 450 °C |
Table I: Ion Transitions for LC-MS/MS Analysis of Ultrashort-Chain PFAS Concurrently with Alternative and Legacy PFAS.
Peak ID |
Compound |
Precursor Ion |
Product Ion |
Internal Standard |
---|---|---|---|---|
1 |
PFPrA |
162.9 |
119.0 |
13C2-PFHxA |
2 |
PFBA |
212.8 |
169.0 |
13C2-PFOA |
3 |
PFPrS |
248.8 |
79.6 |
13C2-PFHxA |
4 |
PFBS |
298.8 |
79.9 |
13C2-PFHxA |
5 |
13C2-PFHxA |
314.9 |
270.0 |
— |
6 |
HFPO-DA |
285.0 |
168.9 |
13C2-PFOA |
7 |
ADONA |
376.9 |
250.7 |
13C2-PFOA |
8 |
PFOA |
413.1 |
368.9 |
13C2-PFOA |
9 |
13C2-PFOA |
415.0 |
370.0 |
— |
10 |
PFOS |
498.8 |
80.0 |
13C4-PFOS |
11 |
13C4-PFOS |
503.0 |
80.0 |
— |
12 |
9Cl-PF3ONS |
530.8 |
350.7 |
13C4-PFOS |
13 |
11Cl-PF3OUdS |
630.7 |
451.0 |
13C4-PFOS |
Results and Discussion
Chromatographic Performance
Analyte peak shapes, retention, and intensity were similar across the reagent water and field water samples. There was a higher baseline for the PFPrA signal in all field water samples (Figure 1), but this did not have a negative impact on peak integration and quantification of PFPrA. No matrix interference was observed for all water samples prepared by two-fold dilution.
Figure 1: Good chromatographic peak shapes and adequate retention were obtained for the simultaneous LC-MS/MS analysis of ultrashort-chain PFAS along with alternative and legacy PFAS in various reagent and field water samples.
80 ppt Reagent Water Standard
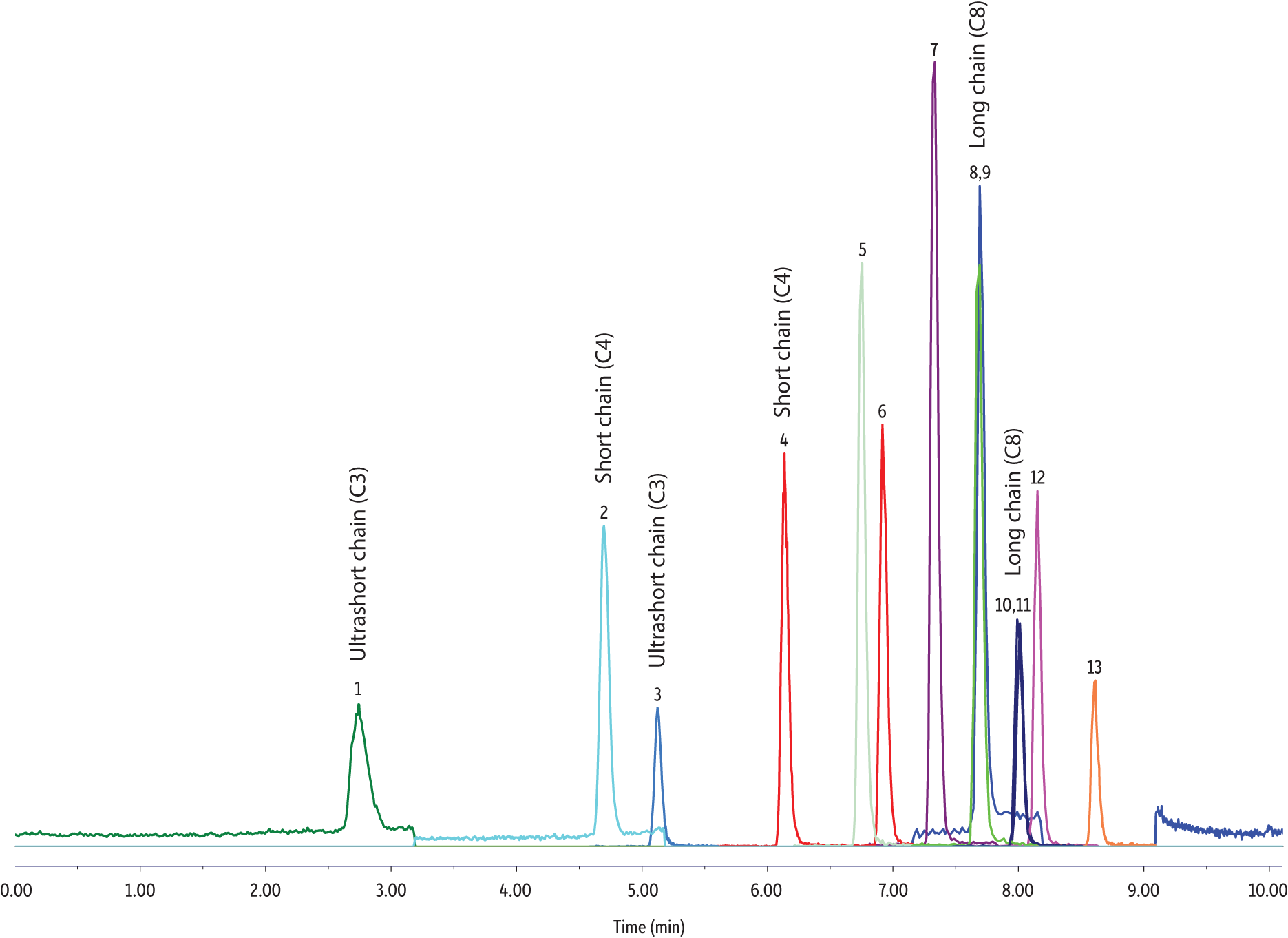
Peaks | tR (min) | Conc. (ng/L) | Precursor Ion | Product Ion | |
---|---|---|---|---|---|
1. | Perfluoropropanoic acid (PFPrA) | 2.74 | 80 | 162.9 | 119.0 |
2. | Perfluorobutanoic acid (PFBA) | 4.69 | 80 | 212.8 | 169.0 |
3. | Perfluoropropanesulfonic acid (PFPrS) | 5.13 | 80 | 248.8 | 79.6 |
4. | Perfluorobutanesulfonic acid (PFBS) | 6.14 | 80 | 298.8 | 79.9 |
5. | Perfluoro-n-[1,2-13C2]hexanoic acid (13C2-PFHxA) | 6.75 | 50 | 314.9 | 270.0 |
6. | Hexafluoropropylene oxide-dimer acid (HFPO-DA) | 6.92 | 80 | 285.0 | 168.9 |
Peaks | tR (min) | Conc. (ng/L) | Precursor Ion | Product Ion | |
---|---|---|---|---|---|
7. | Ammonium 4,8-dioxa-3H-perfluorononanoate (ADONA) | 7.33 | 80 | 376.9 | 250.7 |
8. | Perfluorooctanoic acid (PFOA) | 7.70 | 80 | 413.1 | 368.9 |
9. | Perfluoro-[1,2-13C2]octanoic acid (13C2-PFOA) | 7.70 | 50 | 415.0 | 370.0 |
10. | Perfluorooctanesulfonic acid (PFOS) | 8.01 | 80 | 498.8 | 80.0 |
11. | Perfluoro-[1,2,3,4-13C4]octanesulfonic acid (13C4-PFOS) | 8.01 | 50 | 503.0 | 80.0 |
12. | 9-Chlorohexadecafluoro-3-oxanonane-1-sulfonate (9Cl-PF3ONS) | 8.15 | 80 | 530.8 | 350.7 |
13. | 11-Chloroeicosafluoro-3-oxanonane-1-sulfonate (11Cl-PF3OUdS) | 8.61 | 80 | 630.7 | 451.0 |
80 ppt Fortified Groundwater Sample
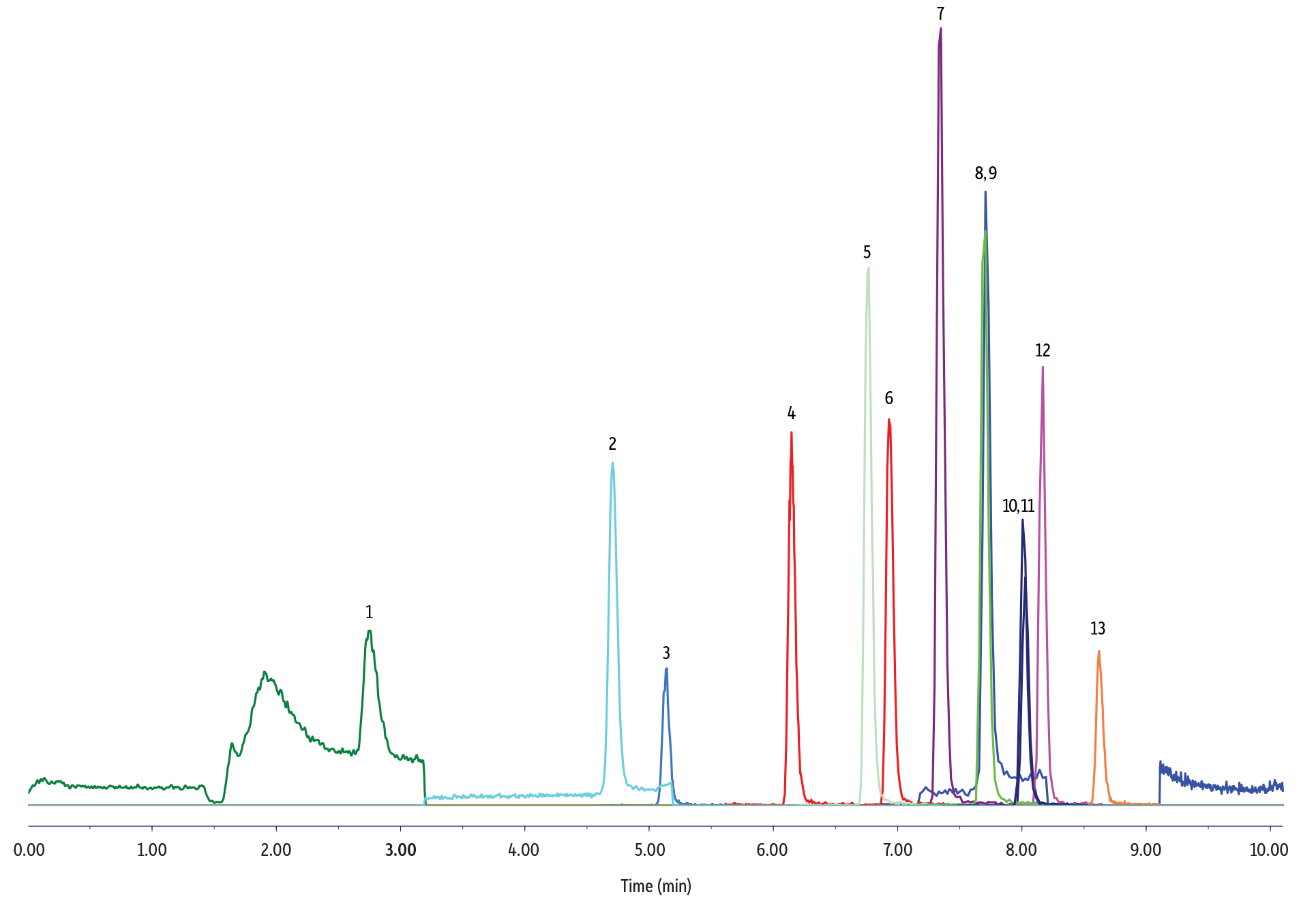
Column | Raptor C18 (cat.# 9304A1E) | ||||||||||||||||||||||||
---|---|---|---|---|---|---|---|---|---|---|---|---|---|---|---|---|---|---|---|---|---|---|---|---|---|
Dimensions: | 100 mm x 3 mm ID | ||||||||||||||||||||||||
Particle Size: | 2.7 µm | ||||||||||||||||||||||||
Pore Size: | 90 Å | ||||||||||||||||||||||||
Temp.: | 40 °C | ||||||||||||||||||||||||
Standard/Sample | |||||||||||||||||||||||||
Conc.: | 80 ppt | ||||||||||||||||||||||||
Inj. Vol.: | 10 µL | ||||||||||||||||||||||||
Mobile Phase | |||||||||||||||||||||||||
A: | Water, 5 mM ammonium acetate | ||||||||||||||||||||||||
B: | Methanol | ||||||||||||||||||||||||
|
Detector | MS/MS |
---|---|
Ion Mode: | ESI- |
Mode: | MRM |
Instrument | UHPLC |
Sample Preparation | In a polypropylene vial, 250 µL of groundwater sample (fortified at 80 ppt) was mixed with 250 µL of 40:60 reagent water:methanol and 5 µL of internal standard solution (5 ng/mL of 13C2-PFHxA, 13C2-PFOA, 13C4-PFOS in methanol). The vial was capped with a polyethylene cap prior to analysis. |
Notes | A PFAS delay column (cat.# 27854) was installed between the pump mixer and the injector. |
Linearity
In this LC-MS/MS method for the simultaneous analysis of ultrashort-chain PFAS along with alternative and legacy PFAS, the calibration range was 10-400 ppt for PFPrA and 5-400 ppt for all other analytes. All compounds showed acceptable linearity with r values ≥ 0.999 and deviations of <20%. 11Cl-PF3OUdS is the only analyte that was quantified using a quadratic regression (1/x weighted) standard curve. All other analytes were quantified using a 1/x weighted linear regression (Figure 2).
Figure 2: Standard Curves.
Accuracy and Precision
The unfortified water samples showed various levels of C3, C4, and C8 PFAS with no detectable PFPrS, ADONA, HFPO-DA, 9Cl-PF3ONS, and 11Cl-PF3OUdS (Table II). For accuracy (%recovery) calculation, the measured amount of analyte in the fortified sample was adjusted based on the unfortified concentration. Table III shows the accuracy and precision results calculated across all three batches of data. Method accuracy was demonstrated by recovery values that were within 20% of the nominal concentration for both fortified sample levels, as well as for the LLOQ concentration standard prepared in reagent water. The %RSD was <15%, indicating acceptable method precision.
Table II: PFAS Concentrations in Unfortified Water Samples.
Detected Concentration (ng/L) | ||||||||||
PFPrA |
PFBA |
PFPrS |
PFBS |
HFPO-DA |
ADONA |
PFOA |
PFOS |
9Cl-PF3ONS |
11Cl-PF3OUdS |
|
Tap Water |
ND |
1.1 |
ND |
ND |
ND |
ND |
ND |
ND |
ND |
ND |
River Water |
ND |
1.6 |
ND |
ND |
ND |
ND |
ND |
ND |
ND |
ND |
Groundwater |
9.0 |
3.4 |
ND |
2.6 |
ND |
ND |
ND |
ND |
ND |
ND |
POTW Water |
11.7 |
10.6 |
ND |
3.0 |
ND |
ND |
15.0 |
6.0 |
ND |
ND |
Table III: Accuracy and Precision.
Conc. (ng/L) |
Average %Recovery (%RSD) | ||||||||
Tap Water |
River Water |
Groundwater |
POTW Water |
Reagent Water |
|||||
10* |
80 |
10* |
80 |
10* |
80 |
10* |
80 |
5** |
|
PFPrA |
96.9 (11.0) |
105 (3.91) |
105 (6.57) |
95.4 (6.84) |
92.0 (9.54) |
99.4 (7.40) |
94.2 (5.29) |
87.2 (8.18) |
103 |
PFBA |
99.3 (9.19) |
108 (1.81) |
108 (5.20) |
110 (1.70) |
104 (8.21) |
108 (6.68) |
108 (8.12) |
97.1 (8.17) |
97.9 |
PFPrS |
100 (4.24) |
107 (3.14) |
103 (6.71) |
105 (2.64) |
105 (8.48) |
109 (6.68) |
109 (5.65) |
103 (9.28) |
99.1 |
PFBS |
101 (5.20) |
106 (1.84) |
99.7 (7.54) |
105 (2.10) |
100 (6.57) |
106 (2.82) |
103 (1.93) |
97.8 (5.85) |
96.0 |
HFPO-DA |
96.2 (7.86) |
102 (4.64) |
96.2 (4.99) |
105 (3.94) |
95.0 (3.59) |
101 (8.92) |
92.9 (4.87) |
90.3 (7.77) |
99.3 |
ADONA |
101 (6.23) |
106 (3.82) |
97.6 (6.36) |
106 (2.32) |
98.4 (2.68) |
105 (4.08) |
98.2 (7.09) |
98.2 (7.09) |
102 |
PFOA |
105 (8.65) |
105 (3.70) |
108 (12.1) |
107 (3.63) |
108 (9.66) |
105 (5.26) |
99.9 (10.5) |
94.5 (7.24) |
100 |
PFOS |
99.3 (2.10) |
108 (4.24) |
112 (1.87) |
107 (4.93) |
101 (2.96) |
102 (2.31) |
104 (4.46) |
98.3 (5.82) |
94.3 (8.85) |
9Cl-PF3ONS |
95.6 (4.60) |
106 (5.93) |
105 (5.37) |
110 (8.20) |
97.2 (4.52) |
107 (7.41) |
101 (6.52) |
99.8 (4.89) |
98.8 |
11Cl-PF3OUdS |
114 (8.78) |
112 (8.91) |
102 (15.0) |
91.5 (2.34) |
96.7 (5.99) |
105 (15.2) |
115 (2.67) |
103 (8.45) |
105 |
*20 ng/L for PFPrA
**10 ng/L for PFPrA
Conclusion
A robust LC-MS/MS method for quantitating a diverse range of PFAS of various chain lengths and structures utilizing a simplified direct injection approach was successfully evaluated in different water sample matrices. Using a Raptor C18 (2.7 µm) 100 x 3.0 mm column with a PFAS delay column, the analytical method was demonstrated to be fast, rugged, and sensitive with acceptable accuracy and precision. This method is suitable for labs needing to report an extended list of PFAS compounds, including the analysis of ultrashort-chain PFAS, for potable and non-potable water testing.
References
- S. Taniyasu, K. Kannan, L.W.Y. Yeung, K.Y. Kwok, P.K.S Lam, N. Yamashita, Analysis of trifluoroacetic acid and other short-chain perfluorinated acids (C2-C4) in precipitation by liquid chromatography-tandem mass spectrometry: comparison to patterns of long-chain perfluorinated acids (C5-C18), Anal. Chim. Acta. 619 (2008) 221-230.
- J. Janda, K. Nodler, H-J. Brauch, C. Zwiener, F.T. Lange, Robust trace analysis of polar (C2-C8) perfluorinated carboxylic acids by liquid chromatography-tandem mass spectrometry: method development and application to surface water, groundwater, and drinking water, Environ. Sci. Pollut.R. 26 (2018) 7326-7336.
- K.Y. Kwok, S. Taniyasu, L.W.Y. Yeung, M.B. Murphy, P.K.S. Lam, Y. Horii, K. Kannan, G. Petrick, R.K. Sinha, N. Yamashita, Flux of perfluorinated chemicals through wet deposition in Japan, the United States, and other countries, Environ. Sci. Technol. 44 (2010) 7043-7049.
- K.A. Barzen-Hanson, J.A. Field, Discovery and implications of C2 and C3 perfluoroalkyl sulfonates in aqueous film-forming foams and groundwater, Environ. Sci. Technol. Lett. 5 (2015) 95-99.